What are DC Shunts
DC shunts are precise, low-resistance components used to measure high direct current (DC). It creates a small voltage drop proportional to the current passing through, which can then be measured by metering devices. Most DC shunts look like this:
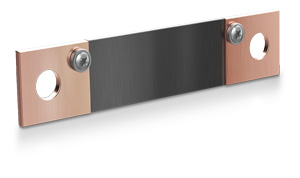
The center piece is made of manganin or constantan, with two terminal strips made of copper. The shunt should be connected in series with a heavy load, such as DC motor, battery charger, or heater. A voltmeter can then be connected to the shunt terminals to measure the voltage drop.
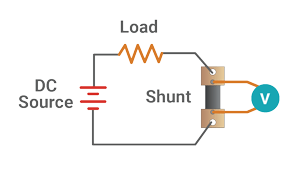
A DC current shunt essentially operates like a resistor with a diminutive resistance that doesn’t affect the normal operation of the other loads. Along with the small resistance, it requires some other special characteristics to function properly.
Working Principles of DC Current Shunts
To understand how DC shunts work, it is important to first understand Ohm’s law, which asserts that the current passing through a conductor is directly proportional to the applied voltage and inversely proportional to the resistance. This relation can be expressed with the following equation:

When a current passes through the shunt, a voltage drop will be created, and according to the above equation, if resistance remains the same, the voltage drop will be directly proportional to the current.
However, the above equation will only describe an ideal scenario, where resistance remains the same. In practice, the resistance of the conductor isn’t always constant. There are many factors that can affect its resistance resulting in a negative impact on the DC shunt’s accuracy.
Temperature
DC shunt must allow a high direct current to pass through causing its temperature to rise. For most metals, the resistance will increase along with temperature, creating a higher voltage drop than it should be.
To solve this problem, engineers chose materials such as manganin (84% copper, 12% manganese, 4% nickel) or constantan (55% copper, 45% nickel) to construct the DC shunts. These alloys have a low-temperature coefficient of resistance (TCR), meaning changes in temperature have minimal effect on their resistance. Using materials such as manganin ensures the DC shunt has high precision over a wide range of temperatures.
As a comparison, the TCR for manganin is 2×10-6 K-1, meaning its resistance increases slightly as the temperature goes up. The TCR for copper is 4.3×10-3 K-1, which is much higher than manganin, meaning the resistance increases significantly with temperature, as shown in the graph below:
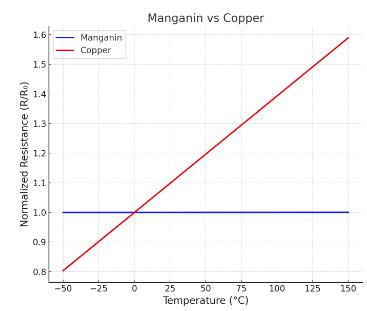
For optimal results, DC shunts should operate within the temperature range of -40°C to 80°C (-40°F to 176°F). Temperature above 140°C will cause permanent change in manganin’s resistance, so proper mounting and cooling system should be implemented to prevent permanent damage to the shunt.
Current Density
DC shunts can come in various models with different rated inputs, ranging from a couple amperes to a few thousand amperes. Towards the end of this spectrum, shunt models with a higher rated input must be made bigger to allow more current to pass through, which leads to a new problem.
The current flowing inside a conductor isn’t always uniform. It will naturally choose the path with the lowest resistance leading to uneven temperature distribution.
This phenomenon will cause higher resistance, lowering the shunt precision level, and in some cases, even raising safety concerns due to uneven heating. Instead of using a single, continuous piece of metal, DC shunts can have a grid design, as shown in the image:
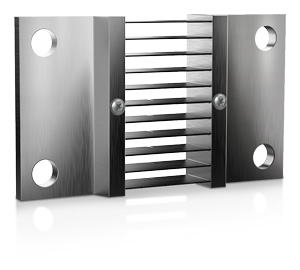
The grid design will force uniform current flow and increase the surface area, which also helps dissipate heat more efficiently.
Applications of DC Current Shunts
DC current shunts play an important role in applications where accurate direct current measurement is required, such as battery management systems, renewable energy systems, electric vehicles and DC motors, public transit systems, and power metering solutions. For example, DC shunts are often used inside electric vehicles to measure the current and regulate the motor speed, torque, and overall performance.
When paired with power and energy meters, DC shunts can provide highly accurate current measurements for monitoring power usage, efficiency, and billing purposes. By delivering accurate, real-time current readings, the DC shunts help energy managers optimize the overall efficiency and performance of the system and prevent current overload, enhancing system reliability and safety.
With so many different types of DC shunts, there are a few ways to choose the right one for a specific application.
How to Choose DC Current Shunts
When selecting a suitable DC shunt, several key factors must be considered, including rated input, voltage drop, accuracy class, and form factor.
Rated Input CurrentCommon rated inputs include 50A, 100A, 200A, 300A, 400A, 500A, 600A, 1000A, and 2000A. Shunts must be able to handle the maximum expected current going through the conductor to be measured, which is based on an appropriate rated input current. The rated input current should be slightly higher than the system’s peak current to ensure safe and reliable operation.
Voltage Drop
The designed output voltage drop must also be taken into consideration. Common output voltages are 50mV, 75mV, and 100mV. Lower voltage drop minimizes the impact on the overall electric circuit but may require sensitive measuring devices.
Accuracy Class
DC current shunts come in various accuracy classes, depending on different applications. A high-accuracy model must be used if the shunt is used for billing or energy metering purposes, where precision is critical.
Form Factor
DC shunt must fit inside the available space. The form factor is also related to the rated current, and high-current shunts are often larger. The required dimensions and mounting options must be verified to ensure compatibility with the overall system design.
Accuenergy offers a line of high-precision DC shunts that can measure DC current at 0.1% accuracy level, offering various input options ranging from 50A to 2000A, with a standard output voltage of 75mV.